Message from the Society President in 2023
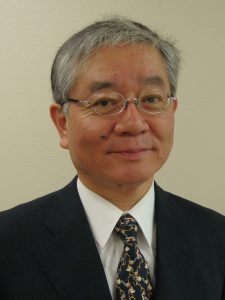
I am writing this message on July 25, 2023. Two months ago, in May, I received a request from the head of the periodicals editorial committee of the Japanese Geotechnical Society (Professor Ito of Tokyo City University) for an article about myself to go into a series of profiles currently being prepared of successive JGS presidents. In my case, I held the office of 30th president for two years, from May 2008 to May 2010. As this was a period that included the society’s changeover of status into a public interest corporation, I had a heavy responsibility to bear in this transition process, and for that reason I could hardly decline. Three of my predecessors, past presidents Ishihara, Ota and Tatsuoka, had already supplied self-profiles, and I was followed in turn by my successors Professor Kusakabe and the current president Professor Koseki. From what I have heard, the total number of requests is planned to come to 11. There is a strict format to follow for the profiles, which makes the writing task far from easy, but the part which most challenged me was section 5, under the heading “About the future of the Japanese Geotechnical Society.” The rubric directions here covered areas such as:
・What future course should the JGS be pursuing?
・The handing down of knowledge and skills
・Advice for young researchers and technicians.
All that a past president can really write about is what they did in their own term as president. The future course of Geotechnology (or of the Geotechnical Society) is not something that can be formulated by past presidents only. But all the same, as it is a matter that I have been ruminating over for the past few days, I will take this opportunity to jot down a few ideas in a haphazard memo-style, fully aware of the randomness that this is bound to lead to.
The coastal ground along the Omaezaki and Hamaoka coast in Shizuoka which slopes down to the sea near where the Hamaoka atomic power plant is sited, is known for its neatly demarcated normal fault alignments, more than a dozen in number and spaced at rather regular intervals of between 50 m and 150 m in alternating layers of consolidated sandstone and mudstone. For safety reasons, there is a need to confirm that this normal fault series has remained inactive since the Quaternary period, or to be more precise, since the late Pleistocene of about 120,000 to 130,000 years ago.
In fact, in the case of these consolidated alluvial layers, we can go back far beyond this confined time scale and affirm that these deposits laid down in the Cenozoic (a part of the Pliocene, 4 or 5 million years farther back in the Tertiary period) have been subjected to a continuous formative process of consolidation over all the millions of years since. Geological surveys of what formed this group of normal faults are well advanced, and there is a wide expert agreement that their origin is not due to seismic activities in the most recent 10,000 years or so of the Holocene. Well, if that is the case, when were they formed, and out of what kind of mechanical background? Eiji Yamada of the Chubu Electric Power Company and a member of the GEOASIA Research Society, took up the hard challenge of researching this question recently under the direction of Professor Toshihiro Noda, and while this may seem to come dangerously close to self-praise, I can only say that the results obtained were outstanding.
These consolidated layers underwent two changes in the course of their prolonged consolidation process. First, ① there was a material change over time in response to changes occurring in the soil skeleton, while at the same time, ② there was also a rise in ground level due to the steeper slope inclination. Through an ongoing numerical analysis of a series of trial-and-error tests, Yamada was able to show that normal faults of this type recurred in places where the slope gradient attained values of just 8 o – 9o. This resulted in a regular succession of parallel and roughly equally spaced fault surfaces, also marked by a reverse domino effect as blocks behind the latest fault face were tipped back. This analysis result offers an uncannily close match to the normal fault configurations actually observed at the Hamaoka site. One is only left to ask: “What other explanation could there be?” In addition to this, there are the behavior characteristics: The appearance of the faults at a particular critical stage in the soil skeleton structure development accounts for the way in which the soft soil layer immediately before this critical point is easily washed away, while just afterward it becomes more consolidated and less vulnerable to damage such as fault slips. Behaviors like these which cannot be explained on the evidence of the surface diagrams alone have always been particularly troublesome for theorists in geomechanics.
Let us pause here, and ask about the significance of these terms “Quaternary” and “Ternary period” and “consolidation” and “diegenetic behavior.” Why do they matter?
Humans are dull creatures, and apt to think that once they have fixed upon names for the phenomena they see in front of them, they have all it takes to understand them. In the Kanto chapter of the Geotechnical Society, it seems that there is now an idea of founding a new committee (with Yoshida Nozomu as committee chair) under the name “Mechanisms of Damage Due to Liquefaction.” I wish them a successful launch. But while it can be said that the committee is now duly named, it is well to realize that the meaning areas covered by these “mechanisms” are still far from completely clear to researchers in Japan, so that in many cases the particular phenomena have to be referred to in long ungainly English terms which may or may not mean much to the specialists concerned. Typical instances are “slaking,” “isotache,” and “cyclic mobility.” But there are others, too. There are also cases of names applied casually “just for use in Japanese” and perhaps without adequate understanding of the meanings or mechanisms implied. “Reliquefaction” is one classic example of this.
To say anything about the Quaternary period, there is a need to know something about the older alluvial and more recent diluvial layers. The diluvial layers are flood deposits going back 2.5 or 2.6 million years and still at a starting stage in terms of consolidation. All that can be said is that they have a high “N value” (resistance to boring) and show no liquefaction history. There seem to have been virtually no investigations of their mechanical properties, let alone of their in situ stress states. Soil mechanics in Japan is held to be the mechanics of “soft (alluvial layer) foundations” and cannot even claim to be treating the soils of the whole Quaternary period. But why should this be so? After all, these Quaternary deposit layers are as widely distributed in Japan as virtually anywhere in the world. Would it be too bold to suggest that the reason behind this paucity of interest might be that Japan acquired its geology and soil mechanics from Europe and America where deposits of this sort were concealed for long ages under glaciers, making Quaternary layers rare in the first place and the research interest in them still rarer?
But let us return to our starting question. Where is geotechnology headed for in Japan?
The various kinds of foundation damage due to earthquakes are just that: variable in kind. Rechristening them a “mechanics of soft foundations” will do nothing to change the fact that they are still under-mapped territory, theoretically speaking. But while it is easy enough to see that this is not a matter that can be wholly resolved with a rethink followed by a renaming, it is nevertheless important to engage in activities that lead beyond this idea of a mechanics of soft foundations.
In geotechnical research undertaken in Nagoya University in 1997 and 1998, an attempt was made to distinguish between “undisturbed” and “remolded” clay through the creation of a concept of “soil skeleton structure.” The difference between “sand” and “clay” soils could then be expressed in terms of a “rate of overconsolidation loss” and a “rate of collapse or decay in the soil skeleton.” This reconceptualization of “soil skeleton structure” and of “overconsolidation” added immensely to the capacity of the previously existing “Cam-clay model,” making it possible for a foundation analysis to provide solutions to almost all the issues involving integrals that had up until then dogged equations of motion, including both the sort arising from supervening earthquake responses and those due to unsaturated conditions in clay soils. But at this this point in our progress, we now find ourselves confronted with the insight that continuous formative actions of soil formation (diegenesis) and consolidation are themselves deeply implicated in the “development of soil skeleton structure” as a total process. How then are these states of “unconsolidated,” “on the way to consolidation,” and “consolidated” to be formulated, not in Japanese or English in particular but in the more general language of elastoplastic mechanics?
Finally – and this is a point I can do no more than glance at in closing – I hear that after achieving splendid results in the alluvial ground, the shield tunneling work for the new linear shinkansen line is now running from one hold-up to the next since reaching the diluvial part that follows. For the past two or three days, the thought has been occurring to me that the elastoplastic soil mechanics of this transition from “unconsolidated” to “on the way to consolidation” might be an important problem to investigate in future research.
Akira Asaoka
Senior research advisor, the Association for the Development of Earthquake Protection (reg. foundation)
Emeritus professor, Nagoya University